Hog Reproduction
thumbnail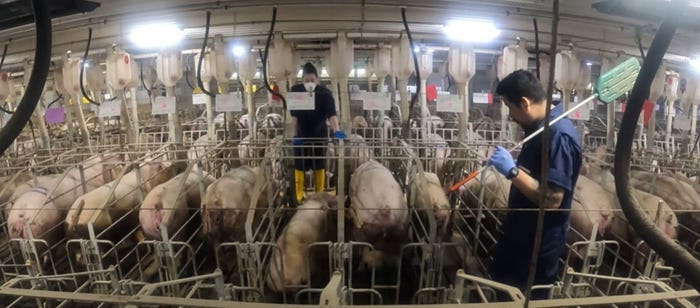
Livestock Management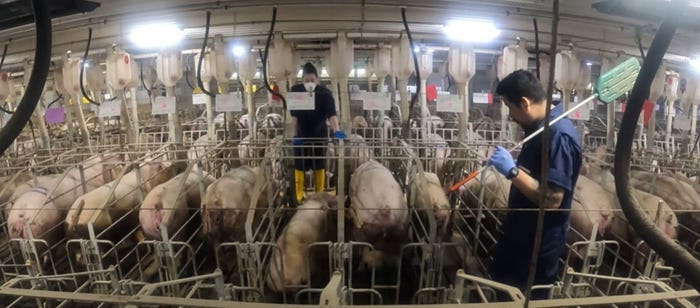
Take a practical approach to reduce sow mortalityTake a practical approach to reduce sow mortality
Iowa Pork Industry Center offers an SOP for caretakers to use while conducting their daily observations.
byAnn Hess