New heat stress index captures complete thermal environment picture
Housed Swine Heat Stress Index will be helpful for designing new ventilation systems and evaluating current ones.
September 20, 2017
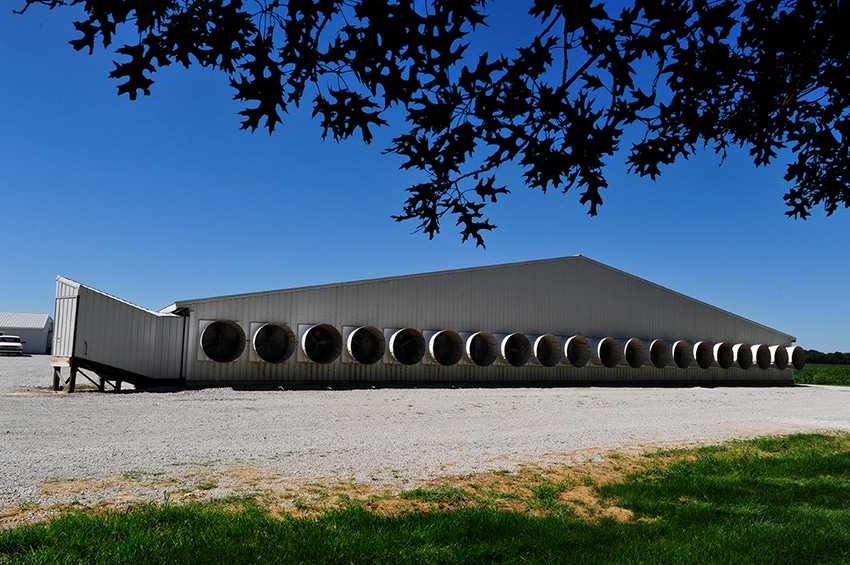
By Brett C. Ramirez, Iowa State University Department of Agricultural and Biosystems Engineering
Many of my previous columns have focused on describing how pigs exchange heat with their surroundings or how our current ventilation systems keep pigs comfortable. None however, have brought the two concepts together. This column will focus on interpreting environmental measurements (e.g., air temperature) into something meaningful. First, we’ll review the heat balance between a pig and its surroundings, then the measurements and methods necessary to describe heat exchange, and conclude with examples of how different environmental conditions can impact pig performance.
Air temperature is not the only parameter that effects how much heat a pig can lose. It is one of four that includes relative humidity, airspeed and surrounding surface temperatures. Each of these parameters describe the different routes a pig can lose heat and together, provide a description of the thermal environment. Each parameter has a different relative impact on the pig’s rate of heat loss. Recall at peak performance, a 155-pound pig produces about 740 BTU (217 W), while a 265-pound pig produces about 932 BTU (274 W) — that is a lot of heat to be dissipated by the pig, ventilation system and surroundings. For example, as air temperature increases, a pig’s heat production decreases in response to re-establishing its thermal balance with the hotter conditions.
Alternatively, air temperature could remain the same, but airspeed increases. As a result, the pig is able to lose more heat and can focus its efforts back to maximum production. So, the question still remains — what combinations of temperature, relative humidity and airspeed are best?
In order to help answer this question, measurements at animal level are best, but if those aren’t possible, estimations will work too (estimating tunnel airspeed by total fan cubic feet per minute divided by cross-sectional area for example). Portable, handheld meters offer a simple and relatively inexpensive solution to obtaining the needed TE measurements. The next step is to combine all these measurements. By no means is this easy and is further complicated by the extensive physiological and behavioral regulation mechanisms available to the pig to cope with its environment. There have been many thermal comfort indices developed to substantially simplify these complex interactions for one selected performance penalty (like respiration rate or feed intake) given different combinations of the TE.
The most predominate and widely used index is the temperature-humidity index, originally developed in the late-’50s for assessing potential heat stress conditions for humans. It has since been adopted for livestock and poultry with many later modifications for different genetic lines, conditions, etc. However, there is only one THI unique for growing pigs and it was developed about 48 years ago for lighter pigs at extreme conditions. Unfortunately, the human THI and corresponding thresholds for normal, alert, danger, emergency conditions are used so prevalently, we have lost touch of the fact that the pig must remain in thermal balance and more than just two parameters effect this. Most importantly for growing pigs, inclusion of body weight is critical because heat production increases (see above) and the surface area to body weight ratio decreases with increasing body weight. Hence, it is impossible to have the same “normal” threshold value (e.g., THI < 74) for a 155-pound and 265-pound pig.
Further, add airspeed in the mix, and now the threshold value goes up. Recall, airspeed increases heat loss; thus, the pig can balance with its environment at a higher THI value. When using THI, it is important to remember its limitations (mainly, it does not include body weight or airspeed).
My solution, after a lot of math and computing, data were generated from a simulation of two theoretical groups of 30 grow-finish pigs — one group at an average body weight of 155 and the other at 265 pounds. Each group was hypothetically exposed to different combinations of air temperature, relative humidity and airspeed (not ideal but much cheaper and faster than experiments). Now we can illustrate how these different parameters influence the pigs. The combined effect of the total TE is summarized by a newly created Housed Swine Heat Stress Index (HS2I), which ranges from 0 (thermally comfortable) to 10 (severely heat stressed), with the intermediate 4 to 6 range representing moderately heat stressed.
Let’s analyze a typical heat stress reduction strategy — elevated airspeeds resulting from tunnel ventilation. For the 155-pound pigs at 85 degree F and 400 cubic feet per minute yields a HS2I of 4 and the 265-pound pig at the same conditions yields a HS2I of 5. Since the larger pigs produce more heat, more airspeed or cooler temperatures are needed to reduce its HS2I to that of the 155-pound pigs. Now, for 155-pound pigs at 85 degrees F and 100 cubic feet per minute a HS2I of 5 results and for 265-pound pigs at these same conditions yield a HS2I of 5.7. For both the 155 and 265-pound pigs, we see that as airspeed increases at 85 degrees F from 100 to 400 cubic feet per minute, HS2I decreases by 1.2, but when airspeed is increased from 400 to 600 cubic feet per minute, HS2I only decreases by 0.55.
Now, let’s look at the scenario if an evaporative pad were to cool the incoming air. We see that HS2I is less than just using tunnel alone — about 1.25 less regardless of airspeed at an ambient air temperature greater than 85 degrees F. Although the air is cooler, there is higher humidity downstream of the pad. If the combined effect of the cooler air temperature and airspeed on heat loss is not sufficient for the pigs and they have to cool themselves with methods that require the evaporation of water (e.g., increased respiration rate or wallowing), they may not be effective and could still cause heat stress. This is shown by a decrease in HS2I from 4.5 to 2.8 when airspeed is increased from 100 to 400 cubic feet per minute when the room air temperature is a cool 75 degrees F (about 86 degrees F outside).
Lastly, the use of sprinklers versus no sprinklers were compared for a tunnel ventilated facility. The evaporation of water directly from the pig’s skin is powerful as shown by a 1.25 decrease in HS2I when water is added at 400 cubic feet per minute. Compared to a 100 cubic feet per minute airspeed with no sprinkling, the 400 cubic feet per minute with sprinkling reduces HS2I by about 3 and moves H2SI from 7.8 to 4.5, indicating a shift from moderately heat stressed to barely heat stressed. Since higher airspeeds
increase the rate of evaporation, we see that HS2I decreases with increasing airspeeds.
Air temperature is not the only parameter that affects a pig’s thermal comfort. It must be combined with airspeed and relative humidity to capture the complete picture. The newly created HS2I does just that — it simplifies the complicated interactions to an easy-to-understand value ranging from 0 to 10. This tool will be helpful for designing new ventilation systems and evaluating current ones.
This work was funded by the Iowa Pork Producers Association and their support is greatly appreciated.
You May Also Like